LNG: The drive for efficiency in increasingly challenging times
Providing reliable, affordable energy to support prosperity and enhance living standards is coupled with the need to do so in ways that reduce impacts on the environment, including the risks of climate change. This is society’s dual challenge and ExxonMobil takes it seriously.
For over 60 years, the LNG Industry has demonstrated and delivered innovation in the delivery of natural gas. The ultimate goal looks to achieve the lowest cost of supply to produce LNG. Two big levers are (1) energy efficiency in operations, and (2) capital cost and execution efficiency. Competitive LNG market trends calls for pulling both levers so that natural gas and LNG can continue to be part of the solution to the Dual Energy Challenge.
Below is a paper recently prepared by Chris W. Sparling, Process Engineering Advisor, ExxonMobil Upstream Integrated Solutions and Don Victory, Chief Process Engineer, ExxonMobil Upstream Integrated Solutions. The paper outlines the trends in “Efficiently building more efficient plants.”
-------------------------------------------------------------
LNG: Trends in energy and execution efficiency
Trends in energy efficiency
Liquefaction of natural gas (LNG) is a highly energy intensive process driven primarily by large refrigeration requirements. Much effort has been applied to increase LNG process efficiency with choices of process components & refrigeration compression drivers. In addition, the industry has also concentrated on how to design and deploy LNG technology for “execution efficiency.” No stone of the LNG value chain is left unturned.
Ultimately LNG competes as a commodity so overall “cost of supply” (COS) is critical to the market share that LNG can obtain and sustain.
Among the technical dimensions considered in designing an LNG liquefaction plant are:
- LNG process efficiency as measured by “specific power,” i.e. the power needed to produce each unit of LNG [1].
- Availability, as the percentage of the year a plant can produce LNG. Availability is largely driven by the choice of refrigeration compressor drivers & their maintenance cycle.
- Capital cost for a unit of production, for example, “capital cost per ton of LNG produced per annum” often expressed as “$ / ton.”
Complementary and symbiotic to the significant innovation in LNG processing is innovation in turbomachinery over the decades. Turbomachinery innovation over this period enabled the growth of LNG plant capacity by enabling more refrigeration capacity from fewer refrigeration compressors. The incentive was perceived to be achieving more process simplicity and “economy of scale” in capital costs.
Turbomachinery used to power the refrigeration that liquefies natural gas has an even longer history of innovation. LNG refrigeration drivers started with steam turbines but soon migrated to gas turbines in order to grow refrigeration and LNG capacity by leveraging improvements from the aviation and power generation sectors. The gas turbine, the workhorse of LNG refrigeration drivers, has risen in fuel efficiency from ~17% to over 40% in ~80 years [2] [3].
That increase in energy efficiency is for a “simple cycle” where the gas turbine is used to directly drive liquefaction refrigeration compressors and or electric generators without further exhaust stack waste heat recovery. Ivan G. Rice tells a fascinating history of the people involved in the development of gas turbines in “combined-cycle” use [4][5]. Combined cycle adds heat recovery from the hot gas turbine exhaust to generate steam which then drives a steam turbine on other turbomachinery. Gas turbine manufacturers have achieved 62% to 64% net efficiency [6][7] on their newest “combined cycle” power plants.
LNG train capacity grew with the development of gas turbines. Gas turbine ‘strings’ were then supplemented using large electric “helper motors” coupled to gas turbines on the same driveline to provide more power for the refrigeration compressors. This increasing refrigeration power in each LNG Train can be described as “power density.”
Another variation on refrigeration drivers can be seen with two baseload LNG plants which exclusively use large electric motors to power baseload production of “all-electric LNG.” The prize of “all electric LNG” is higher annual availability & LNG production because gas turbine scheduled maintenance is removed from directly impacting LNG production. Conventional wisdom has been that “all-electric LNG” came at a higher capital investment. Technology is available to be more energy efficient in LNG production. It would be nice if such energy efficiency could be realized without a capital investment increase which directionally offsets energy efficiency in the total LNG cost of supply.
Trends in overall energy to produce LNG
Overall energy efficiency can be benchmarked by the total energy for natural gas liquefaction divided by the LNG produced. Of course, the better the energy efficiency then the lower the CO2 intensity and Green House Gas (GHG) emissions for the produced LNG. This table shows the general trend of decreasing energy consumption per MTA of LNG produced. These numbers are illustrative for a cross-section of industry processes & turbomachinery combinations and of course venture specifics will dictate which may be the proper combination for any particular project.
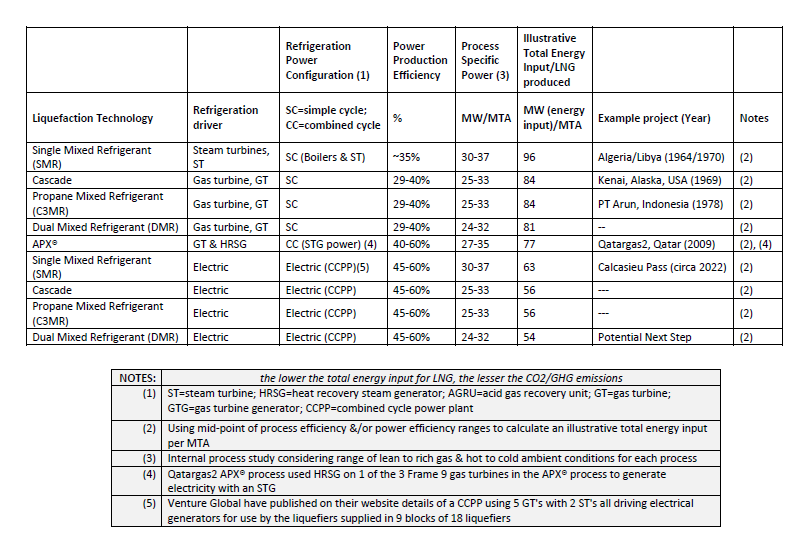
Trends in execution efficiency
The overall cost of a project is a function of the cost of its process equipment & turbomachinery yet is usually dominated by direct and indirect costs associated with bulks such as piping, steel, cabling, and concrete not to mention construction support infrastructure. As LNG practitioners, we need to understand the tradeoffs & inflection points in execution which lead to capital cost. The importance of the designer’s sensitivity to avoid inadvertently driving up bulks and capital cost can be seen if one references spiking capital costs indices like IHS Markit’s Upstream Capital Cost Index (UCCI).
Over the decades process efficiency as well as its associated “intensification” and execution efficiency have gone hand in hand. Process intensification tends to directionally improve execution efficiency. More process service is accomplished while constructing less tonnage of facilities. Usually “bulks” for installation are reduced with an attendant reduction in work hours. In addition process intensification tends to move labor hours to an offsite fabrication facility where productivity is higher.
In LNG a good example of process intensification, while also achieving process and execution efficiency is the evolution in liquefaction heat exchange:
- Brazed aluminum heat exchanger ‘cores’ or ‘blocks’ can be packaged together in factory assembled ‘cold boxes’ along with associated piping and instrumentation in a compact “assembly.”
- Brazed aluminum heat exchangers can also be installed in vessels in an arrangement often referred to as “core in kettle” or “blocks in vessel.” Further, multiples of these vessels can be assembled with interconnecting pipes and associated instrumentation within a cold box.
- Spiral wound heat exchangers have over the years been manufactured in increasing size thus decreasing equipment count. These exchangers can also be instrumented and insulated before arriving at the installation site.
The above technical evolutions to increase process efficiency, the train capacity and the power density of LNG plants by utilizing larger gas turbines and packaged heat exchange equipment directionally tends to decrease the amount of site labor associated with the liquefaction equipment. But it may inadvertently drive bulks (e.g. pipes and fittings) and labor costs higher in other “code of accounts” of project execution.
“Modularization” is a long-used execution technique whereby projects construct ‘building blocks’ of the overall process at external sites with subsequent hook up and commissioning using less labor at the project site. We can illustrate modularization by picturing a creation assembled out of LEGO® bricks as ‘building blocks.’ A modularization execution approach is typically selected after a cost/benefit analysis for doing the work in the multiple locations relative to location labor rates and productivities, cost of field overheads, cost of construction support infrastructure, etc.
ExxonMobil examined multiple modularized LNG projects and learnings from non-LNG onshore and offshore modular projects. The general themes that emerged from this review were published along with proposed guidance for future modularized LNG projects [8].
Once a module is designed it may be repeatedly manufactured without re-doing the engineering, a classic “Design One. Build Many!” (D1BM) approach used on LNG, FPSO, & oil-sands projects for decades. As an example, the AP-X® LNG trains referred to in the table are the largest LNG trains built after 60 years of increasing baseload LNG train capacities and were repeated D1BM across 6 LNG trains in 3 projects.
In contrast, a “manufacturing” of LNG capacity can be seen in a project that took “Final Investment Decision” (FID) based on 18 D1BM mid-sized “liquefiers” that cumulatively may produce 10 MTA of LNG at one site [9]. This scheme represents a break with the decade’s long trend of increasing LNG “train” sizes.
Reducing cost and cost risk in execution
A “question” behind efforts for innovation and cost reduction should be “Can we reduce quantities?” as quantities drive total labor.
One conventional wisdom is that putting process facilities into modules costs more since it adds quantities such as structural steel. This may be generally true though often offset to a degree in savings in other direct cost categories (concrete, piping). Further, by “Design One. Build Many!” in a factory-type setting, there may be enhanced labor productivity and fewer direct hours since a trained work force will be working in a production mode. Finally, owners of facilities value both qualitatively and quantitatively the economic benefit of a lower risk of site costs “blowing out” on the high side. This is a particular concern executing a project in a remote location where skilled labor may be in short supply and the supply chain may not keep the site productive. With proper system design and execution planning the debits of increased “packaging” may offset the cost of “stick-building” in a remote location.
Second, directing work to where labor can be more productive may entail reduced indirect costs pertaining to infrastructure investment. Building remote LNG plants may require camps, temporary fabrication facilities, even in some cases material offloading facilities, roads, bridges, and an airport. Incorporating prefabricated components may save in site infrastructure investments.
And third, total labor and work could be minimized with proper value engineering. This can be most readily appreciated by the minimization of engineering hours for liquefiers that are D1BM.
Integrating “Manufactured LNG” and “All-electric LNG” for energy and execution efficiency
Let’s consider a thought exercise involving LNG liquefier “manufacturing” and D1BM module execution tactics extended out to near 100 units. Such brings to mind Henry Ford’s Model T manufacturing efficiencies which made automobiles affordable for many families.
Manufacturing LNG liquefier components could involve:
- Producing identical units in a controlled environment using the same knowledgeable labor pool to deliver higher manufacturing efficiency.
- Full quality control and pre-commissioning at the manufacturing site to further limit site hours & staffing.
- The combined offsite manufacturing efficiency with reduced site work-hours & staff count delivering a lower capital cost versus other execution alternatives.
Achieving a cost benefit from manufacturing LNG liquefier components will depend on the achieving a target manufacturing cost, avoiding carry over of work to the production site where the components are installed, and controlling field work and productivity for hook-up and commissioning. Chris Caswell's paper at LNG 2019 "Does (Train) size matter?" elaborates on the topic of "economy of scale" versus "economy of (manufactured) units" [10]. He notes that return data is not yet available on the latter execution approach and that in all cases an assured execution plan is essential. Is it too early to declare if manufacturing of LNG liquefiers components is a trend of the future? Perhaps. Analogs suggest it be considered under some circumstances.
Small and mid-sized LNG plants have long used electric motors for the refrigeration compressor as the electrical grid provides adequate stability for their starting power. To date two large baseload “all-electric” LNG plants are operating with large electric motors on refrigeration drivers.
Electric motors for liquefaction refrigeration can range from less than a megawatt to up to 75 MW. The selection of electric motor size needs to balance liquefaction equipment count with site-wide electrical and process system dynamics & stability. The need for motor-start or speed control provisions balances with process & electrical system stability within the cost implications identified above. Selection of the liquefaction process, its refrigeration compressor(s), and its driver electric motor size largely sets the rest of the manufactured LNG liquefier components. All must be fit into overall site-wide electrical and process system dynamics & stability.
There is potential to power some or all of the LNG liquefaction compression and ancillaries using purchased power. LNG that is developed near a well-established power grid may be able to purchase power generated from renewables or nuclear, rather than, or to supplement power produced on site. The objective is to lower the total cost of LNG supply and to lower emissions. The lower the effective price to power LNG liquefaction, the lower the cost of the produced LNG.
Another option to render an “all-electric LNG” project is having a site-dedicated combined cycle power plant (CCPP) either operating as a “power island” or connected “to the grid” to supply refrigeration power. A site “power island” can be designed and built using power industry specifications versus, for example, API specifications.
There are multiple options for achieving combined cycle type efficiencies and lower CO2 emissions for a unit of produced LNG. Gas turbines can be fitted with waste heat recovery to generate steam to drive steam turbines whether in a power island or if used directly to drive refrigeration compressors.
Best energy and execution efficiency
Considering the above collectively it may be possible to directionally gain more energy & capital efficiency simultaneously via implementation of a “manufactured LNG” scheme composed of:
- Proper selection of a matched “power island” with sets of LNG liquefiers that are manufactured and site assembled after due consideration of site-wide operating dynamics and analysis of capital and operating costs structures.
- Adopting a dual mixed refrigerant cycle such as ExxonMobil’s Enhanced Mixed Refrigerant (EMR®) process [11] for the sets of LNG liquefiers.
EMR® produces about 5-15% more LNG for the same power than a single mixed refrigerant process so should achieve a lower total power and CO2 emission per unit of LNG produced. Note that EMR® also requires smaller average piping sizes for refrigerant suction lines than a typical single mixed refrigerant process of the same power compressor. That should make EMR® more amenable for manufacturing and site assembly at lower quantities and work hours.
Conclusion
2020 has certainly been an unprecedented year for our global community. The year began with financial challenges in the energy markets and was further strained after March 11, when the World Health Organization declared the COVID-19 outbreak a pandemic. This global health crisis rapidly turned into an economic crisis as well.
Eventually, we will get through these challenges. In the longer term, however, we will still face the dual challenge of meeting the growing demand for energy while at the same time, reducing the environmental impacts. More efficiently produced LNG will provide an energy option on the road to resolving the dual challenge.
ExxonMobil’s global LNG operations.
REFERENCES:
[2] https://www.powermag.com/a-brief-history-of-ge-gas-turbines-2/
[3] https://www.powermag.com/the-power-interview-what-drove-the-gas-turbine-technology-leap-at-ge-over-the-past-70-years/
[4] https://www.turbomachinerymag.com/the-evolution-of-the-combined-cycle-power-plant-i/
[5] https://www.turbomachinerymag.com/the-evolution-of-the-combined-cycle-power-plant-ii/
[6] https://www.powermag.com/efficiency-improvements-mark-advances-in-gas-turbines/
[7] http://www.mhps.com/news/20200402.html
[8] “ExxonMobil adopts new approach for design of modularized onshore LNG export plants.” Ashley Guy, Sorin Lupascu, Donna J. Parker, Don Victory and Greg Henke, LNG Journal, March 2016, pages 38-42
[9] http://venturegloballng.com/calcasieu-pass/facility/#.XnkikKhKiUk
[10] https://www.gti.energy/wp-content/uploads/2019/10/200-LNG19-05April2019-Caswell-Christopher-presentation.pdf
[11] “ExxonMobil Enhanced Mixed Refrigerant (EMR) process for next generation LNG projects.” Denton R D, McMahon P D, Vines M J. Paper presented at the 90th Annual Gas Processors Association Convention, San Antonio, TX, USA, 3-6 April 2011.
Why ExxonMobil?
- Our long-standing, global experience in every aspect of the world of LNG, means we can help our customers get maximum benefit from using LNG and natural gas.
- Our agile approach helps us create progress quickly, with the flexibility to work on everything from small to large-scale projects.
- We use structured finance and commercial creativity to deliver successful solutions that work best for our customers.
- We work with the world’s best LNG technology and engineering providers to identify and help develop everything they need to get the most out of using LNG.
- Building a long-term relationship with our customer helps us to understand your needs better, working with you to deliver a clean, reliable source of energy for communities, businesses, and industry
Our Experience
- ExxonMobil has been a pioneer & champion of innovation in the LNG industry with a long history of innovations with our partners that goes back to the birth of he LNG Industry in the 1960’s.
- We researched, patented and, in some cases, licensed more efficient LNG processes (eg; our High Pressure Expander Process (HPXP®) we reported on recently & our ExxonMobil Enhanced Mixed Refrigerant (EMR®) process)
- We utilize a structured methodology for executing our projects and we have both a project Layout Center of Excellence and a Modularization Center of Excellence to provide expertise & guidance in decisions for how to execute LNG projects for the lowest cost of supply.
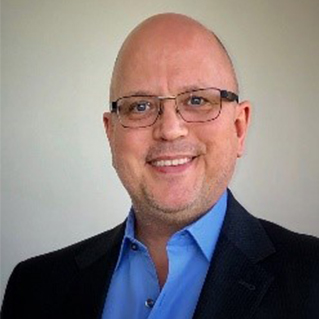
Chris W. Sparling, Process Engineering Advisor, ExxonMobil Upstream Integrated Solutions
Chris has 30 years of experience in engineering, operations, technology commercialization & global project execution, including commissioning, startup & facilities debottlenecking. He’s held positions of increasing responsibility including Project Engineer, Engineering Manager and Advisor on assignments in Canada, Qatar, USA, Japan, Indonesia and France on projects from conventional oil and gas through LNG and Canadian Oil sands. On two occasions, Chris has been loaned to ExxonMobil’s partners to support their project execution with the most recent being the Coral South FLNG project, operated by Eni, as Facilities Lead for the Liquefaction topsides modules.
Chris earned a BSc degree in Chemical Engineering from The University of Alberta, in Canada, in 1990.
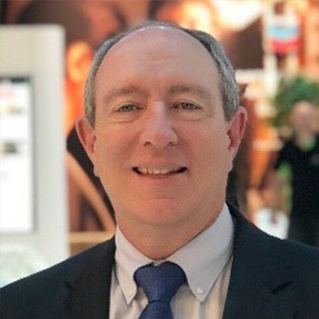
Don Victory, Chief Process Engineer, ExxonMobil Upstream Integrated Solutions
Don has 39 years of process experience in research, operations, engineering, and project management, had assignments in Malaysia and Russia, and has supported numerous international gas development projects. From 2009 to 2018 Don led the engineering team responsible for process design, safety engineering, and conceptual engineering for ExxonMobil’s major upstream projects including business lines as diverse as LNG and heavy oil. Don was co-founder of the company’s Layout Center of Excellence and Cryogenics Center of Excellence.
Don earned a BSc degree in Chemical Engineering from The University of Connecticut in 1981.